Summary
The ACtIon4Cooling project is making use of existing data, algorithms, models and adaptations to propose monitoring capabilities of different Solar Radiation Management (SRM) approaches, including Stratospheric Aerosol Injection (SAI), Marine Cloud Brightening (MCB) and Cirrus Cloud Thinning (CCT). The project is investigating natural analogues, the building of novel datasets and analysing study cases for the assessment of potential SRM deployments. Synergistic measurements of volcanic aerosols, marine clouds affected by ship-track emissions and aviation-relevant cirrus clouds will also be studied.
ACtIon4Cooling is an ESA-funded project of the German Aerospace Center (DLR), National Observatory of Athens (NOA ReACT) and University of Leipzig aiming at indoor research on Solar Radiation Management (SRM).
Background
The project addresses a critical scientific and policy challenge: understanding and quantifying aerosol-cloud interactions (ACI) to reduce uncertainties in climate projections and assess the feasibility, risks, and governance of solar radiation modification (SRM) technologies as a supplementary climate intervention strategy.
ACtIon4Cooling tackles the persistent uncertainties in effective radiative forcing due to aerosol-cloud interactions, particularly those related to aerosol-induced modifications of cloud micro- and macrophysical properties.
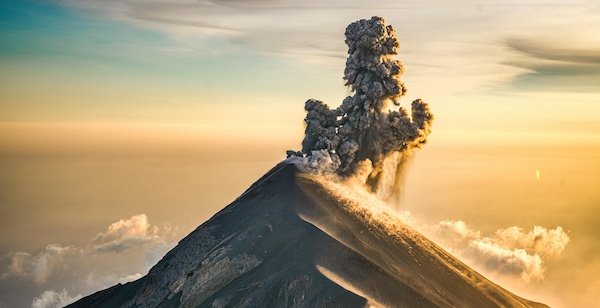
Despite decades of research, estimates of total aerosol forcing still vary by over 50%, largely due to limitations in observing and modelling aerosols and their interactions with clouds. Key challenges include insufficient vertical resolution in passive satellite observations, poor characterisation of cloud condensation nuclei (CCN) and ice-nucleating particles (INP), and retrieval dependencies among cloud properties.
Integrated, high-resolution, and vertically-resolved aerosol and cloud measurements, using both passive and active remote sensing, are required to constrain ACI processes. A better understanding of these interactions is essential not only to quantify current climate drivers but also to evaluate the potential of SRM approaches such as stratospheric aerosol injection (SAI), marine cloud brightening (MCB), and cirrus cloud thinning (CCT).
ACtIon4Cooling aims to contribute to the emerging discourse on SRM as a potential emergency or transitional response to rapid climate change, particularly if mitigation efforts fail to meet the Paris Agreement targets. While SRM methods could provide rapid cooling by increasing Earth's albedo, they also pose significant scientific, environmental, and geopolitical risks, including stratospheric ozone depletion, shifts in precipitation patterns, and ethical concerns over governance and deployment. The project seeks to inform responsible SRM research through improved scientific evidence, particularly on the timing, regional effects, and unintended consequences of such interventions.
By advancing the observational and theoretical understanding of ACI, the ACtIon4Cooling supports both climate science and policy. It aims to reduce critical uncertainties in climate modelling, evaluate the physical plausibility and climate impacts of SRM technologies, and provide evidence-based input for governance frameworks on the responsible research and potential deployment of geoengineering solutions.
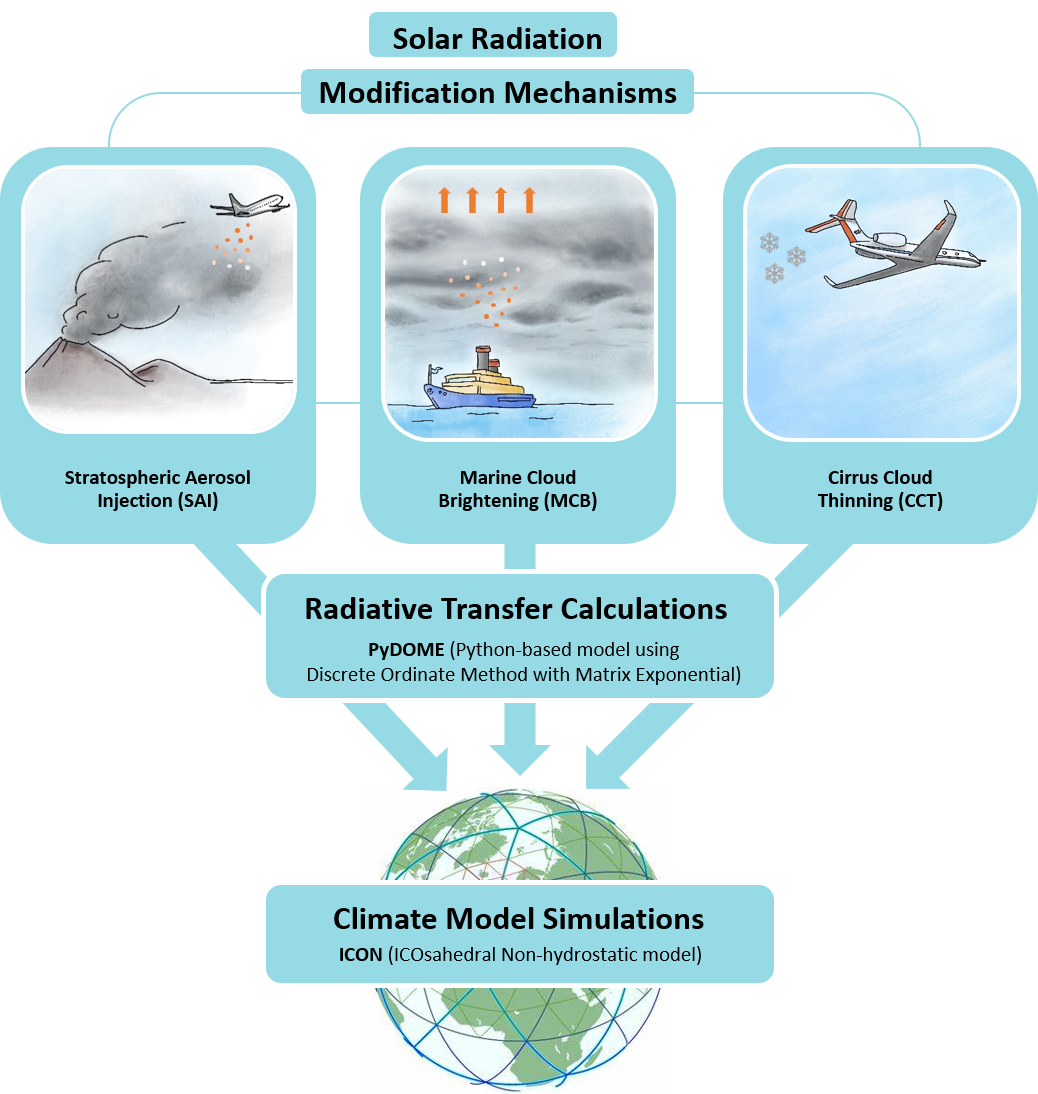
ACtIon4Cooling aims to advance our understanding of aerosol-cloud interactions (ACI) and their implications for climate forcing and geoengineering strategies. Its primary scientific objectives include:
- Enhancing Measurement and Retrieval Techniques:
The project seeks to improve the observational capabilities for aerosols and clouds by integrating high-resolution, vertically-resolved remote sensing data from both passive and active instruments. This is intended to overcome current limitations in cloud masking and aerosol retrieval, particularly in terms of capturing critical microphysical properties such as cloud droplet number concentration (Nd), cloud optical thickness (COT), and liquid water path (LWP). - Reducing Uncertainties in Radiative Forcing Estimates:
A key goal is to minimize the large uncertainties—over 50% spread—in estimates of aerosol-induced effective radiative forcing. This will be achieved by refining models of aerosol absorption and the processes governing CCN and ice-nucleating particles, thereby providing a more accurate quantification of aerosol-cloud interactions and their impacts on cloud albedo and lifetime. - Supporting Solar Radiation Modification (SRM) Research:
The project is designed to generate robust scientific evidence to assess the feasibility, timing, and potential efficacy of various SRM approaches, including stratospheric aerosol injection (SAI), marine cloud brightening (MCB), and cirrus cloud thinning (CCT). By elucidating the mechanisms that control aerosol-induced adjustments in cloud properties, the project will help evaluate how these SRM techniques could alter local and global climates, particularly in terms of mitigating rapid warming and extreme weather events. - Informing Policy and Governance:
Beyond its scientific pursuits, the project aims to contribute to the policy discourse on geoengineering by providing detailed assessments of the environmental risks and governance challenges associated with SRM deployments. This includes evaluating potential negative side effects, such as ozone depletion and unintended alterations of regional climate regimes, to support the development of comprehensive, evidence-based guidelines for responsible SRM research and implementation.
The first phase focuses on data acquisition and preparation. This involves collecting satellite (e.g., CALIPSO, EarthCARE, Aeolus, TROPOMI) and ground-based (e.g., ACTRIS, BSRN, AERONET) observations to characterise natural analogues such as volcanic eruptions (e.g., Pinatubo, Calbuco) and ship-track aerosols. These datasets provide essential inputs to constrain microphysical and optical properties of aerosols and clouds relevant to SRM analogues.
The second phase consists of data exploitation and analysis, where advanced radiative transfer modelling (using tools like DOME and pyDOME) will simulate radiation fields at the top and bottom of the atmosphere. These simulations will incorporate realistic aerosol and cloud properties, validated against satellite and ground-based measurements. Special attention will be given to the vertical profiling of aerosols and clouds, particularly in the stratosphere and marine boundary layer.
The third phase focuses on development and validation of SRM observational proxies. This includes investigating satellite-derived metrics (e.g., aerosol layer height, UV absorbing aerosol index) and their sensitivity to SRM-relevant perturbations. The impact of aviation-induced cirrus modification will also be analysed using lidar-based polarization data (PLDR) and linked to cirrus cloud formation processes.
The fourth phase is dedicated to sensitivity studies and scenario simulations. The radiative impact of SRM scenarios will be tested under a range of atmospheric conditions using advanced sensitivity analyses and phase function corrections. These outcomes feed into global and regional climate simulations using the ICON atmospheric model, aligned with GeoMIP/CMIP6 protocols, to evaluate broader climatic consequences such as temperature anomalies, teleconnection shifts, and extreme weather impacts.
A final integrative activity will synthesise all results, facilitating the development of monitoring and attribution requirements for a dedicated SRM satellite mission. Cross-cutting actions include validation campaigns (e.g., PANGEA/ASKOS) and the assessment of uncertainties associated with SRM interventions.
Overall, ACtIon4Cooling combines high-resolution observations, radiative transfer theory, and climate modelling to build a robust scientific basis for assessing the feasibility, effectiveness, and risks of SRM strategies.
Project lead
DLR Remote Sensing Institute, Atmospheric Processors Department (DLR MF-ATP)
- WP10X lead (Project management and Outreach)
- WP 200 Lead (Scientific Requirements, Impact Analysis Assessment)
- WP401 Lead (Marine Cloud Brightening)
- WP402 Lead (Observation Synergies &Radiation Closure)
Project partners
DLR Physics of the Atmosphere Institute, Lidar Department (DLR PA-LID)
- WP403 Lead (Cirrus Cloud Thinning)
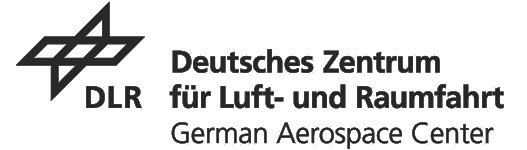
National Observatory Athens, Remote Sensing of Aerosols, Clouds and Trace Gases (NOA ReACT)
- WP300 Lead (Coordination with EU projects)
- WP400 Lead (Aerosol/Cloud Properties)
- WP402 lead (Stratospheric Aerosol Injection)
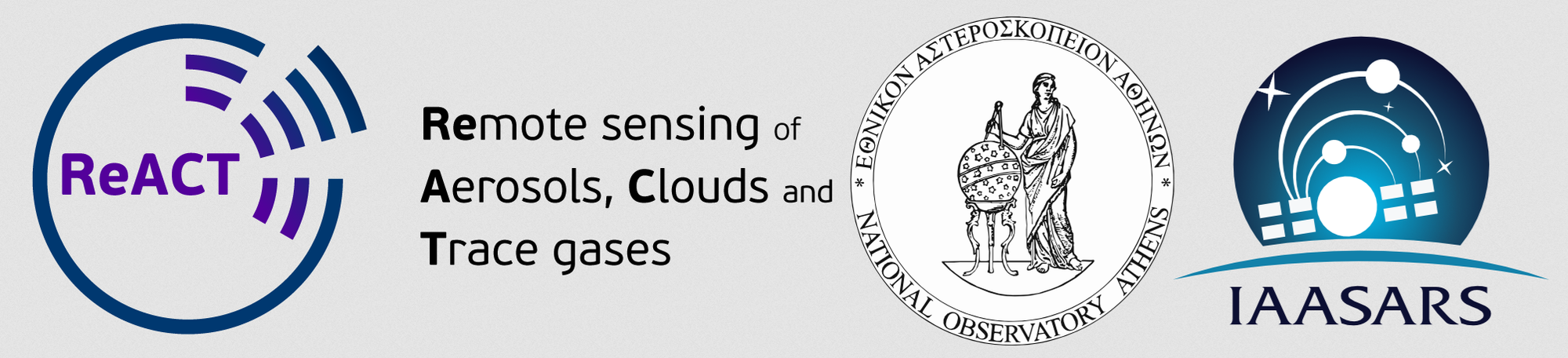
Universität Leipzig - Faculty of Physics and Earth System Sciences
- WP500 Lead (Scientific Synthesis)
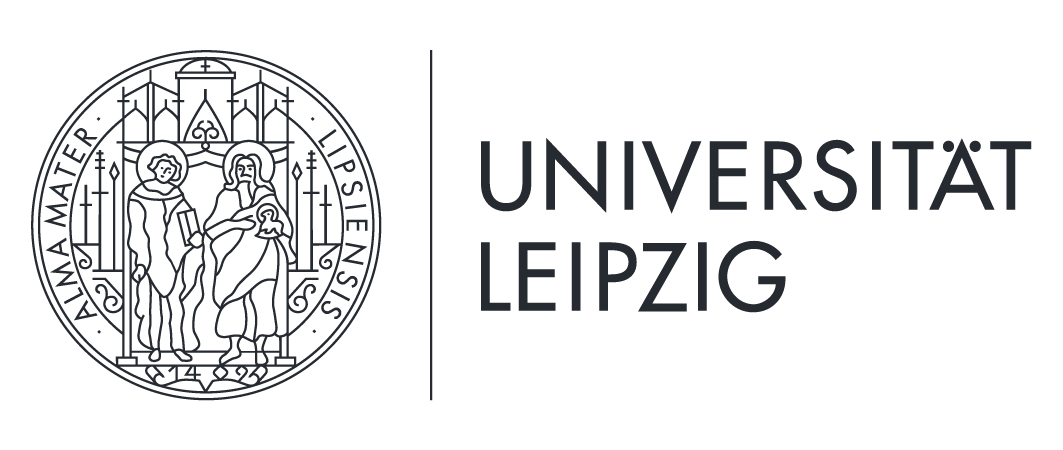
Science Leader: Dr. Athina Argyrouli (DLR)
Project Leader: Dr. Pascal Hedelt (DLR)
ESA Technical Officer: Dr. Michael Eisinger
LinkedIn page: https://www.linkedin.com/groups/10061777/
Overview
Stratospheric Aerosol Injection is a technique which aims at increasing the backscattering of solar radiation directly back into the space to cool Earth's climate by injecting Aerosols in the Earth’s stratosphere.
The quantity and nature of the aerosols in Earth's stratosphere determine the degree of cooling or warming and affect the course of important chemical processes in the stratosphere. In ACtIon4Cooling we will study the impacts of aerosols in the stratosphere, related to Stratospheric Aerosol Injection (SAI), using natural analogues of volcanic aerosols injected in the stratosphere after volcanic eruptions. Detailed measurements for the derivation of their microphysical and optical properties is essential for the quantification of their radiative effect, as well as model evaluation and understanding of the processes that take place.
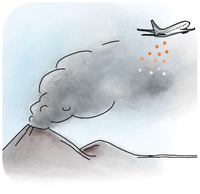
Stratospheric Aerosol Injection - the benefits and options
There is a body of evidence that suggests SAI has potential as an effective technique in reversing the Earth’s warming rapidly. Satellite observations after major volcanic eruptions have shown a global cooling impact following the release of large concentrations of reflective particles into the lower stratosphere. Due to their chemical composition, the net radiative effect of volcanic aerosols is negative because they more effectively scatter shortwave solar radiation in comparison to absorbing longwave terrestrial radiation.
The addition of sulphate particles into the stratosphere after a volcanic eruption provides a natural analogue for Solar Radiation Modification (SRM) deployment: The Mount Pinatubo eruption, in 1991, injected approximately 20 million tons of SO2 into the stratosphere - as measured by the Total Ozone Mapping Spectrometer (TOMS) -and the SO2 cloud remained in the atmosphere for weeks (Bluth et al., 1992). The global annual-mean cooling in the following two years was quantified at 0.3-0.5°C and coincided with a reduction of the global water vapour concentrations. This demonstrated that the water vapour feedback in the climate models is crucial for making climate change projections (Soden et al., 2002).
For the deployment of a SAI approach, a scaling up of a global mean temperature reduction of about 1-2°C would require the annual continuous injection rates of several million tons of sulphur dioxide equivalent to the injection of SO2 concentrations after Mount Pinatubo volcanic eruption.
Currently, the technology to achieve injection aerosol precursors at a predefined altitude of the stratosphere is lacking. There are however a few climate geoengineering proposals (Vaughan and Lenton, 2011) and review studies on capabilities and costs on such an SRM deployment (Smith and Wagner, 2018).
Although the injection of volcanic aerosols is widely used as the natural analogue of SAI, it may not be the optimum solution due to its adverse effects, such as stratospheric ozone depletion. For this reason, the ACtIon4Cooling project will investigate simulated cases of SAI, with varying microphysical and optical properties. The size distributions will be similar to volcanic aerosols, but the project will take into account larger and smaller particles, to investigate the effects of quicker or slower deposition of the particles, respectively. The refractive index will be that of calcite particles, which have been reported to not have an effect on ozone depletion (e.g. Tilmes et al., 2022) and will have a non-spherical (spheroidal) shape.
The ACtIon4Cooling project will investigate the eruptions of Mnt. Pinatubo, Calbuco, Eyjafjallajökull and Mnt. Etna, using space and ground-based remote sensing observations to derive the microphysical and optical properties of volcanic particles injected in the stratosphere. Special focus will be given to the use of lidar observations (from space and ground), along with their synergy with passive remote sensing observations, to acquire valuable information on particle injection height, along with their microphysical and optical properties. The observations will be acquired at variable locations (i.e. Iceland, Ruang/Indonesia, Chile, Philippines) and seasons (i.e. winter, spring and summer), from a large period of time, as this is available from the observational datasets for each eruption case.
References
Bluth, G. J. S., Doiron, S. D., Schnetzler, C. C., Krueger, A. J. and Walter, L. S. Global tracking of the SO 2 clouds from the June1991 Mount Pinatubo eruptions. Geophysical Research Letters. 19: 151–154, https://doi.org/10.1029/91GL02792,1992.
Soden BJ, Wetherald RT, Stenchikov GL, Robock A. Global cooling after the eruption of Mount Pinatubo: a test of climate feedback by water vapor. Science. Apr 26;296(5568):727-30, https://doi.org/10.1126/science.296.5568.727, PMID: 11976452, 2002.
Vaughan, N.E., Lenton, T.M. A review of climate geoengineering proposals. Climatic Change 109, 745–790. https://doi.org/10.1007/s10584-011-0027-7, 2011.
Tilmes, S., Visioni, D., Jones, A., Haywood, J., Séférian, R., Nabat, P., Boucher, O., Bednarz, E. M., and Niemeier, U.: Stratospheric ozone response to sulfate aerosol and solar dimming climate interventions based on the G6 Geoengineering Model Intercomparison Project (GeoMIP) simulations, Atmos. Chem. Phys., 22, 4557–4579, https://doi.org/10.5194/acp-22-4557-2022, 2022 a.
Overview
This potential climate intervention technique modifies the albedo of the low clouds over water by introducing cloud condensing nuclei-effective aerosols to produce a larger amount of smaller in size cloud droplets that reflect more sunlight back into space. Latham et al. (2008) estimated that a 6% increase of the albedo of marine stratocumulus clouds can offset the warming caused by the CO2 doubling.
A natural analogue for this solar radiation modification deployment comes from ship exhaust aerosol particles directly entering marine stratocumulus clouds (Christensen and Stephens, 2011) altering cloud microphysical and macrophysical properties. Cloud albedo responses to ship tracks depends on several parameters such as the mesoscale cloud structure, the free tropospheric humidity, and cloud top height (Chen et al., 2012).
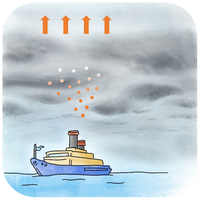
Marine Cloud Brightening - the benefits and options
The ACtIon4Cooling project will evaluate the effectiveness in producing measurable cooling of the Earth system - at global and regional scale - resulting from MCB deployment.
The project will analyse marine clouds that are co-located with ship-track signatures over the Mediterranean Sea (and elsewhere): Changes in cloud cover and reflectivity of those marine clouds with ship emission signature will be evaluated.
Marine cloud properties will come initially from the spaceborne Ultraviolet Visible Near-infrared (UVN) spectrometers, such as TROPOMI on Sentinel-5 Precursors (Veefkind et al., 2012). The TROPOMI operational algorithms for the retrieval of cloud parameters (Loyola et al., 2018) make use of Earth-shine reflectance measurements in the spectral windows of UV, VIS and NIR. Complementary information for the marine clouds captured by TROPOMI instrument will be exploited from VIIRS on Suomi-NPP. The MCB aerosol information is captured by the TROPOMI sensor in the Oxygen absorption bands (i.e., Aerosol Layer Height), and in the UV spectral window as well. In particular, the ultraviolet (UV) Absorbing Aerosol Index (AAI) is widely used as an indicator for the presence of absorbing aerosols in the atmosphere (Kooreman et al., 2020; Torres et al., 1998a). The ship-track signature of aerosols in the TROPOMI cloud retrievals will be investigated via the scientific NASA TropOMAER (TROPOMI aerosol algorithm), which simultaneously retrieves aerosol optical depth (AOD), single-scattering albedo (SSA), and the qualitative UV aerosol index (UVAI) (Torres et al., 2020).
Validation of the MCB dataset is foreseen for the marine clouds captured by PANGEA (PANhellenic GEophysical observatory of Antikythera) observatory at the island of Antikythera (35.861N, 23.310E, 110m a.s.l). PANGEA is an active member of ACTRIS and AERONET network and a satellite Cal/Val center in the Mediterranean region. Validation of the MCB dataset will be strengthened from the ESA funded experimental campaigns (e.g. the ASKOS experiment). Finally, the ESA EarthCARE mission (Illingworth et al. 2015, Wehr et al., 2023), supported by the complementary datasets of the NASA PACE mission (Werdell et al., 2019), offers the opportunity to exploit novel parameters of the aerosol-cloud system.
References
Veefkind, J.P., I. Aben, K. McMullan, H. Förster, J. de Vries, G. Otter, J. Claas, H.J. Eskes, J.F. de Haan, Q. Kleipool, M. van Weele, O. Hasekamp, R. Hoogeveen, J. Landgraf, R. Snel, P. Tol, P. Ingmann, R. Voors, B. Kruizinga, R. Vink, H. Visser, P.F. Levelt, TROPOMI on the ESA Sentinel-5 Precursor: A GMES mission for global observations of the atmospheric composition for climate, air quality and ozone layer applications, Remote Sensing of Environment, Volume 120, Pages 70-83, https://doi.org/10.1016/j.rse.2011.09.027, 2012.
Loyola, D. G., Gimeno García, S., Lutz, R., Argyrouli, A., Romahn, F., Spurr, R. J. D., Pedergnana, M., Doicu, A., Molina García, V., and Schüssler, O.: The operational cloud retrieval algorithms from TROPOMI on board Sentinel-5 Precursor, Atmos. Meas. Tech., 11, 409–427,https://doi.org/10.5194/amt-11-409-2018, 2018.
Kooreman, M. L., Stammes, P., Trees, V., Sneep, M., Tilstra, L. G., de Graaf, M., Stein Zweers, D. C., Wang, P., Tuinder, O. N. E., and Veefkind, J. P.: Effects of clouds on the UV Absorbing Aerosol Index from TROPOMI, Atmos. Meas. Tech., 13, 6407–6426, https://doi.org/10.5194/amt-13-6407-2020, 2020.
Torres, O., Bhartia, P. K., Herman, J. R., Ahmad, Z., and Gleason, J.: Derivation of aerosol properties from satellite measurements of backscattered ultraviolet radiation: Theoretical basis, J. Geophys. Res., 103, 17099–17110, https://doi.org/10.1029/98JD00900, 1998. Torres, O., Jethva, H., Ahn, C., Jaross, G., and Loyola, D. G.: TROPOMI aerosol products: evaluation and observations of synoptic-scale carbonaceous aerosol plumes during 2018–2020, Atmos. Meas. Tech., 13, 6789–6806, https://doi.org/10.5194/amt-13-6789-2020, 2020.
Illingworth, Anthony J., H. W. Barker, A. Beljaars, Marie Ceccaldi, H. Chepfer, Nicolas Clerbaux, J. Cole et al. "The EarthCARE satellite: The next step forward in global measurements of clouds, aerosols, precipitation, and radiation." Bulletin of the American Meteorological Society 96, no. 8 (2015): 1311-1332, https://doi.org/10.1175/BAMS-D-12-00227.1, 2015.
Latham, J., Rasch, P., Chen, C.-C., Kettles, L., Gadian, A., Gettelman, A., Morrison, H., Bower, K., and Choularton, T.: Global temperature stabilization via controlled albedo enhancement of low-level maritime clouds, Philos. Trans. Roy. Soc. London, 366, 3969–3987, https://royalsocietypublishing.org/doi/10.1098/rsta.2008.0137, 2008.
Overview
Cirrus Cloud Thinning is a technique which aims at reducing the amount of cirrus clouds in the sky. Cirrus clouds affect the escape of infrared radiation back out to space and so contribute to the net heating of Earth's atmosphere. CCT techniques involve the seeding of cirrus clouds with ice-nucleating particles to increase the sedimentation rate of the ice crystals.
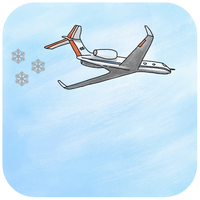
Cirrus Cloud Thinning - the benefits and options
Aviation exhaust emissions release greenhouse gases, particles and water vapour into the atmosphere. At high altitudes this can create linear clouds called contrails and cirrus clouds. These clouds add to cloud cover and indirectly modify properties of existing cirrus.
There may be no perfect natural analogues for CCT, but aviation-relevant cirrus cloud and the involved processes can help to understand how CCT might work in the real atmosphere. Within ACtIon4Cooling, we will use the existing airborne observations to focus on specific clouds where aviation emissions are dense and further derive their optical thicknesses and ice crystal number concentrations. From a statistical perspective, the project will also use satellite data to determine the optical and microphysical properties of cirrus clouds as a function of latitude and longitude as input for Earth system model studies.
The CCT technique is not well studied to date. Gasparini and Lohmann (2016) performed climate model simulations and concluded that cirrus cloud seeding cannot result in a significant cooling due to the large uncertainties of the complex microphysical mechanisms of those clouds. Penner et al., 2015 found that the cirrus cloud seeding cannot be considered as a viable climate intervention technique but they associate their conclusion to the uncertainties in the modelling and observations of cirrus clouds and particularly, the balance between homogeneous and heterogeneous ice nucleation.
Cirrus Cloud Thinning (CCT) Analogues
There are several best-known natural analogues of CCT: Volcanic eruptions inject sulfur dioxide and water vapor into the atmosphere, forming sulfate aerosols and affecting cirrus formation indirectly. During a mineral dust episode (like Saharan dust), a significant amount of mineral dust is lifted into troposphere, which may act as INPs. The consequent transport of dust particles, depending on meteorological factors, from their source regions across large distance will spread the influence into larger scales. Aircraft-emitted particles may also act as INPs, causing heterogeneous nucleation in regions with a favorable atmospheric state. It leads to the formation of contrails and exerts indirect effects on the existing cirrus clouds. In the frame of the current project, we will focus on the changes of cirrus cloud properties responding to aviation impact as a natural analogue of CCT.
Previous studies indicated that the enhanced heterogeneous nucleation caused by aviation exhaust particles can be responsible for the high values of PLDR of cirrus clouds (Urbanek et al., 2018; Li and Groß, 2021, 2022). Furthermore, cirrus clouds with enhanced PLDR exhibit larger effective ice particles and lower number concentrations (Groß et al., 2023). The findings provide strong support that changes in microphysical properties of cirrus clouds depending on aviation emissions can serve as a natural analogue of CCT.
References
Gasparini, B. and Lohmann, U. Why cirrus cloud seeding cannot substantially cool the planet. Journal of Geophysical Research: Atmospheres. 121: 4877–4893, https://doi.org/10.1002/2015JD024666 2016.
Groß, S., Jurkat-Witschas, T., Li, Q., Wirth, M., Urbanek, B., Krämer, M., Weigel, R., and Voigt, C.: Investigating an indirect aviation effect on mid-latitude cirrus clouds – linking lidar-derived optical properties to in situ measurements, Atmos. Chem. Phys., 23, 8369–8381, https://doi.org/10.5194/acp-23-8369-2023, 2023.
Li, Q., and Groß, S.: Changes in cirrus cloud properties and occurrence over Europe during the COVID-19-caused air traffic reduction, Atmos. Chem. Phys., 21, 14573-14590, https://doi.org/10.5194/acp-21-14573-2021, 2021.
Li, Q. and Groß, S.: Satellite observations of seasonality and long-term trends in cirrus cloud properties over Europe: investigation of possible aviation impacts, Atmos. Chem. Phys., 22, 15963–15980, https://doi.org/10.5194/acp-22-15963-2022, 2022.
Penner, J. E., Zhou, C. and Liu, X. Can cirrus cloud seeding be used for geoengineering? Geophysical Research Letters. 42: 8775–8782, https://doi.org/10.1002/2015GL065992, 2015.
Urbanek, B., Groß, S., Wirth, M., Rolf, C., Krämer, M., and Voigt, C.: High depolarization ratios of naturally occurring cirrus clouds near air traffic regions over Europe, Geophys. Res. Lett., 45, 13,166–13,172, https://doi.org/10.1029/2018GL079345, 2018.
Latest news & events
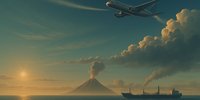
ACtIon4Cooling & STATISTICS Workshop | 17 June
ACtIon4Cooling & STATISTICS Joint SRM Workshop
Meer leren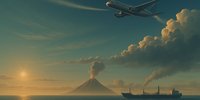
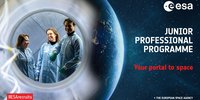
New Position: Junior Professional in AI for Climate Science
Opportunity for early-career Artificial Intelligence talent to join ESA’s Actionable Climate Information Section
Meer leren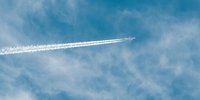